The following research topics are currently being explored by the team:

T1 mapping and concentration measurement of T1 diagnostic and theranostic agents
​
Since clinical imaging protocols generally do not include imaging sequences for T1 mapping, the team is interested in developing approaches to generate quantitative T1 images from T1-weighted anatomical imaging sequences such as TSE, VIBE or MPRAGE sequences, which are themselves widely used for patient diagnosis.
Obtaining these quantitative T1 maps is of particular interest in the diagnosis of neurodegenerative diseases, where T1 variations are an imaging marker in many conditions such as multiple sclerosis. These T1 maps can also be used to quantify the impact or the concentration of diagnostic or theranostic T1 agents in vivo. For certain key applications, such as dynamic contrast-enhanced perfusion imaging, a quantitative assessment of T1 changes due the contrast agent is required to obtain maps of blood volume, blood flow or mean transit time in tumors. Similarly, in the case of theranostic agents that combine diagnostic and therapeutic functions, it is necessary to quantify their concentration in order to assess their therapeutic efficacy. These concentration measurements are based on changes in tissue T1 relaxation time values after administration of these T1 agents.
This research topic of the team is currently being pursued as part of the Ph.D. project of Audrey Lavielle.
The figures below illustrate the approach developed by the team. From left to right, (i) design of a phantom with a physiological range of T1 and T2 values, (ii) plot of phantom T1 values as a function of the image signal intensity, (iii) native T1-weighted image and derived T1 map, (iv) 3D view of the contrast agent concentration map in brain metastases.
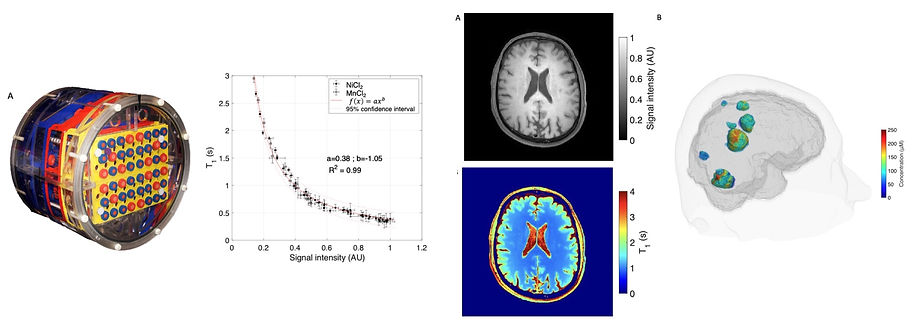


​
Inhaled contrast agents
​
For several years, the team has been interested in the administration of MRI contrast agents via the airways by instillation, aerosol or nebulization. One of the motivations for developing these approaches is to be able to diagnose and eventually treat pulmonary pathologies (asthma, pulmonary fibrosis, lung cancer) using the airways as a direct access route to lung tissue. A second important application of MRI contrast agent delivery to the lungs is the ability to image the biodistribution of aerosolized or nebulized drugs. The efficiency of airborne particle deposition in the lung (penetration, reproducibility, distribution, fraction deposited) remains an active research topic in which MRI can play an important role due to its high spatial resolution and the absence of ionizing radiation.
A current research project of the team in this area involves imaging the pulmonary distribution of surfactants administered to premature infants to treat respiratory distress syndrome. This research topic is currently being pursued as part of the Ph.D. project of Oumaima Marfouk.
These two images below were obtained after nebulization of a surfactant solution and a Gd-based contrast agent in a rabbit lung. Left: maximum intensity projection (MIP) image, right: segmentation of the central airway (blue) and peripheral lung (red).


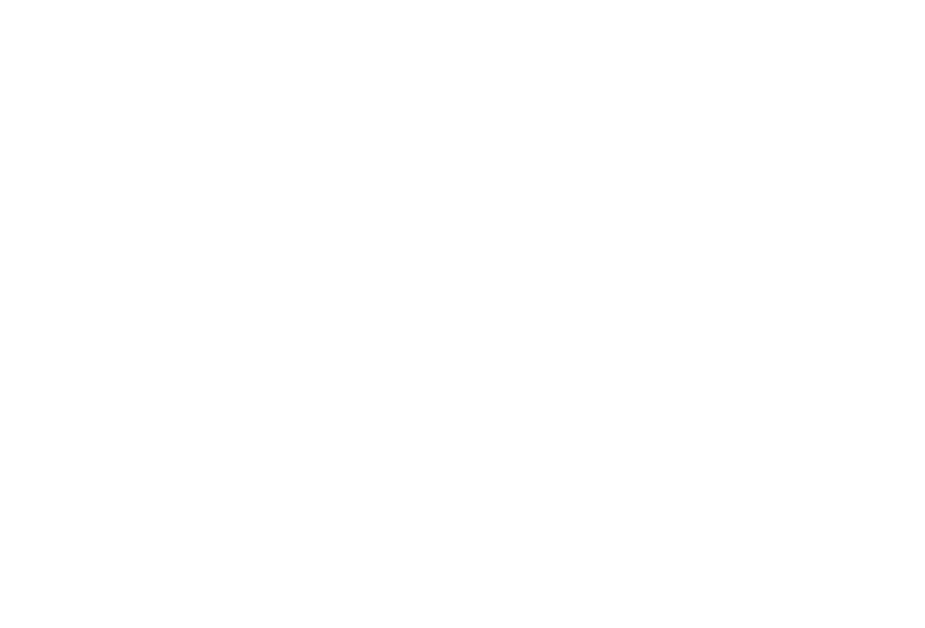
​
From microcoils to implanted theranostic devices
​
In recent years, the team has developed miniaturized instruments for MRI and MRS. First, we worked on external microcoils for real-time MRS analysis of cerebral dialysate composition. We then developed implantable microcoils to study tumor metabolism in situ and image tumor tissue with very high spatial resolution. Current developments focus on the use of hybrid theranostic implantable devices consisting of an NMR microcoil for diagnosis and a capillary for delivery of therapeutic solutions by convection enhanced delivery (CED) into pathological tissue. This research direction was largely explored during the thesis of Justine Deborne, defended in 2022 (https://hal.science/tel-03875872v1).
The following figures summarize the instrumental development and application of the implanted theranostic device. From left to right, (i) photo of the device with microcoil and CED capillary, (ii) proton spectra and high-resolution MRI images in healthy brain and brain tumor obtained with the implanted device, (iii) changes in concentration of metabolites after administration of antitumor molecule.
​
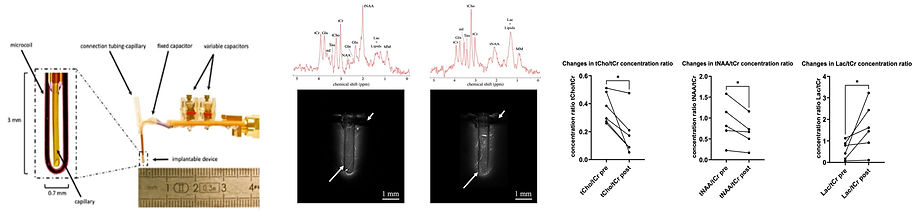


​
MRI-guided intracerebral metal chelation
​
In this area of research, we are interested in using MRI to evaluate the efficacy of metal chelation techniques in the brain.
Iron, zinc, copper or manganese ions are involved in many essential mechanisms (oxygen transport, electron transfer, cellular metabolism, neurotransmission) to name a few. Other metals can accumulate in the body due to environmental exposure to pollutants such as lead, mercury or manganese. Dysregulation of the balance of these ions is thought to adversely affect the survival of brain cells and, in particular, certain populations of neurons, as evidenced by numerous degenerative diseases, including Alzheimer's and Parkinson's. Regulating the concentration of these metal ions in the brain is therefore crucial, and their chelation and elimination from the body is one of the most promising approaches.
MRI has an important role to play here, both as an established technique for determining the concentration of MRI-detectable metal ions (Fe2+/3+, Mn2+) and as a means of assessing in vivo the impact of chelation techniques on brain tissue and its functionality. This research topic is currently being pursued as part of a collaborative research project supported by the French Agence Nationale de la Recherche (ANR).
The images below illustrate some aspects of this research activity of our team. From left to right: (i) chelation of Mn2+ ions using convection-enhanced delivery (CED) of a chelating solution in the brain, (ii) Follow-up of a polymer-based chelating fiber inserted in the brain.
​
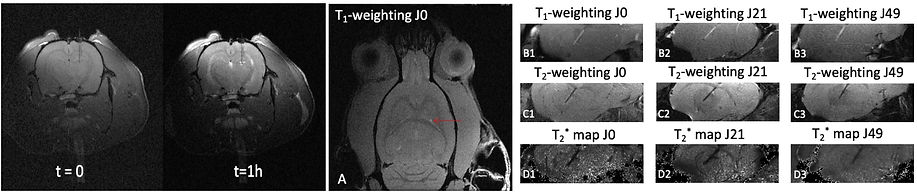
